Heat exchanger design with additive manufacturing

Written by nTop
Published on August 18, 2022
Additive manufacturing enables you to create next-generation heat exchanger designs to meet increasing product requirements. This article guides you through the engineering design techniques you need to know and how to get started.
Heat exchangers (HEX) are crucial in many heat transfer applications, from cooling electronics to recuperating heat in industrial facilities. These devices are essential for thermal management as it ensures the product and processes perform as intended over their expected lifespan, and they are critical in energy production.
However, for many high-performance applications, we have reached the limit of what is technically possible using traditional manufacturing methods in terms of heat exchanger efficiency or size. This is where additive manufacturing technologies come into the picture. The design freedom of additive manufacturing allows you to create more innovative designs and empowers you to optimize heat exchanger performance.
This article explores how you can use additive manufacturing to develop more efficient and compact heat exchangers.
Additive manufacturing for heat transfer
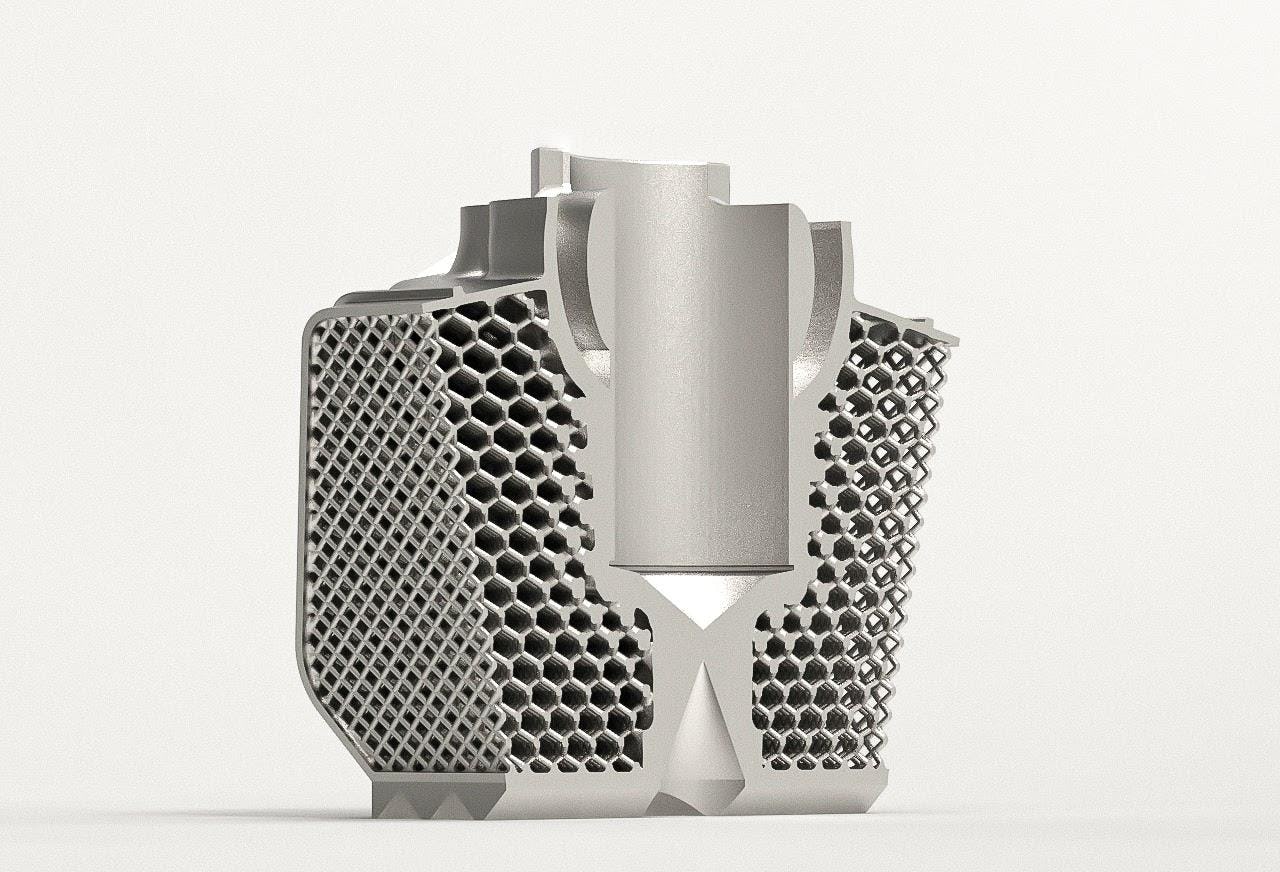
Combustion engine cylinder heat sink of UAV drone designed by Cobra Aero, made from AlSi10Mg, an aluminum alloy.
The additive manufacturing process enables you to create a range of optimized geometries to increase energy efficiency, system performance, and heat transfer. These new capabilities mean you can create novel additively manufactured heat exchanger designs featuring optimized internal geometry for varying heat transfer and flow conditions.
Here are some of the key benefits that additive manufacturing can offer for thermal management and heat transfer applications:
- Construct intricate Triply Periodic Minimal Surface (TPMS) lattices, which are ideal for designing heat exchanger cores — allowing you to pack the maximum surface area into the smallest volume possible while fulfilling size, weight, performance, and manufacturability requirements.
- Apply new shape optimization techniques to achieve specific performance requirements, like pressure drop or flow rate, and design highly optimized structures that would be impossible to manufacture with traditional means.
- Fabricate heat exchangers in one piece. By consolidating an assembly into a singular component, you can minimize the potential and common regions of failure or leakage, increasing the overall reliability of the design. This also reduces inventory and labor costs.
Basics of heat exchanger design
To better understand how 3D printing can substantially impact HEX design, let's take a closer look at the essential components of a heat exchanger.

The anatomy of an additively manufactured heat exchanger.
The makeup of each heat exchanger may differ, but the basic layout of each design is the same. Here are some important design considerations for the essential elements when designing heat exchangers for additive manufacturing.
The body
The shape of a heat exchanger depends on the application and available design space. The traditional pill, ovular, or plate setups still provide high performance, but with additive manufacturing, you now have more freedom to explore a greater range of heat exchanger body shapes. For example, you can design heat exchangers with external dimensions that conform to the available irregular space or are embedded inside the structure of load-bearing components.
The core
The core of the heat exchanger is typically filled with a lattice structure. Additive manufacturing is uniquely capable of producing these complex structures. TPMS structures, like the gyroid or diamond, yield the best results for liquid-to-liquid heat exchangers because they provide a large area for heat transfer and naturally separate the flow into domains. Beam-based lattices also find applications in one-domain solid-to-liquid or solid-to-air heat exchangers.
Piping and plenums
Inlet and outlet piping and plenums gradually introduce the flow into the heat exchanger and act as a pressurized buffer zone. Their geometry can be optimized using CFD data, engineering intuition, and expertise to distribute the flow evenly and reduce pressure drop.
Baffles
Baffles are necessary to prevent cold and hot flows from mixing, which are typically introduced at the entrance and exit of the core. Another option is to create "virtual" baffles that stir the flow without completely blocking it off by locally changing the lattice thickness or cell size.
Goals of heat exchanger design
There are generally three goals that you attempt to achieve when optimizing heat exchanger designs.
Maximize heat transfer
Products are becoming increasingly mechanical and electrical, which means they require greater heat dissipation. Improving a heat exchanger's heat transfer coefficient leads to better thermal management. The heat transfer rate is proportional to the available heat transfer area. Increasing the surface area of the heat exchanger core leads to an increase in the heat transfer coefficient. You can achieve this result in two ways — either by increasing the overall size of the heat exchanger (which is not always an option) or by adjusting the core’s geometry.
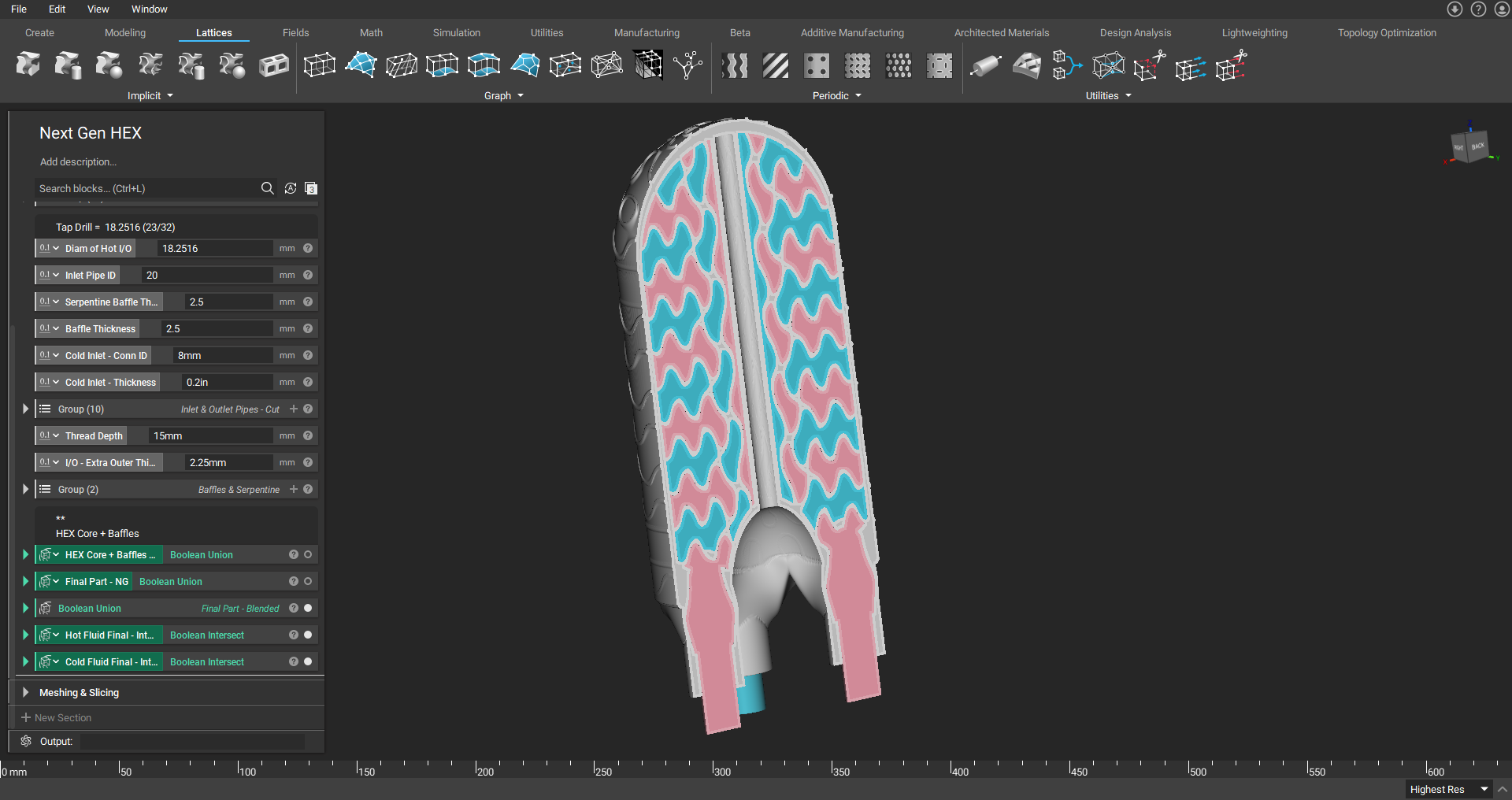
Cross section of a fuel-cooled oil cooler optimized for additive manufacturing. The hot and the cold fluid domains are visible.
Minimize pressure drop
Increasing the complexity of the core often leads to higher pressure drops between the inlet and the outlet of the heat exchanger. A higher pressure drop means you need a larger pump to drive the system. However, this is not always an available option due to space or cost constraints or because you are replacing an existing heat exchanger on a system designed for a specific pressure drop.
You can use various techniques to ensure that pressure remains consistent throughout the heat exchanger. Creating a uniform flow distribution, introducing flow guides, and manipulating the lattice core are effective ways to minimize the pressure drop.

Cold plate with gyroid flow guides for EV inverter designed by Puntozero, manufactured in an unalloyed aluminum powder.
Minimize size and weight
You may be facing several size, weight, and shape constraints when designing your HEX. For example, you may have the same amount of space in your product for the HEX but need increased heat transfer. Or you might want to embed some heat transfer functionality, like cooling channels, in the structural components.
You might also encounter other limitations. The space available could be irregular, or the cost of additive manufacturing could be proportional to the material used, which means you need to reduce weight to keep the cost of your AM HEX within budget. Perhaps your HEX is going on a vehicle, so the weight must be as low as possible. All of these scenarios can necessitate a weight and size reduction.
However, AM HEX design goals are often in opposition to each other — reducing weight often reduces surface area, lowering the heat transfer coefficient. Traditional manufacturing techniques limit your ability to develop lightweight designs while meeting other requirements, like heat transfer, strength, and reliability. The design freedom of AM enables you to manage your product requirements concurrently, so you can minimize size and weight without compromising your other goals.
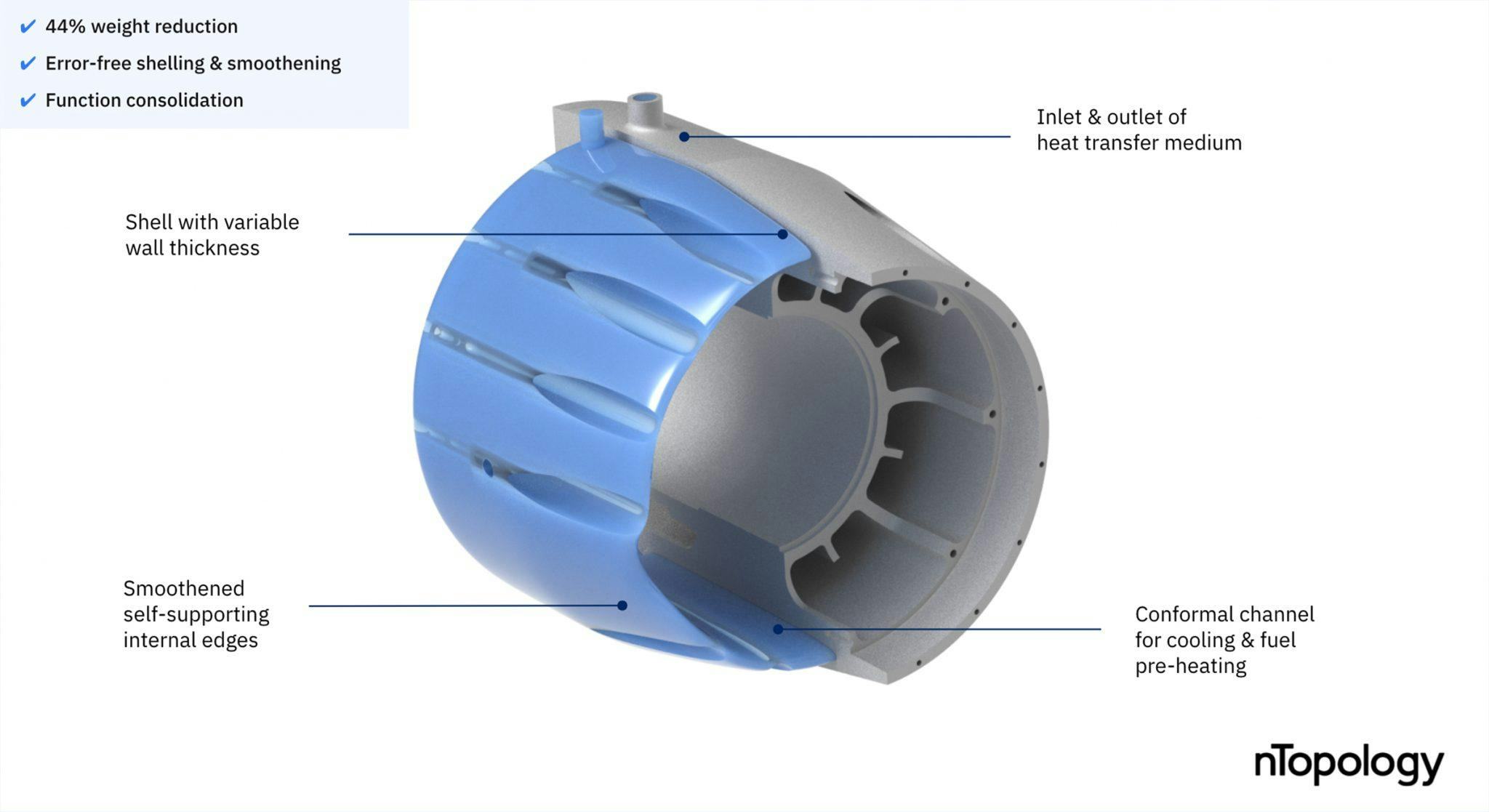
KW Micro Power created a shell to reduce the weight of the casing of their generator. The empty shell was used as a channel for cooling liquid, creating a compact heat exchanger.
Manufacturing processes and materials
Metal Powder Bed Fusion (M-PBF)
Metal powder bed fusion is a commonly used 3D printing technique that involves using a laser or electron beam to melt and fuse the material powder. You can use the following methods in heat exchanger design: direct metal laser sintering (DMLS), electron beam melting (EBM), and selective laser sintering (SLS).
With MPBF methods, you can manufacture lighter heat exchangers that require less space and feature complex geometries and maximized surface area. These methods enable the production of 3D shapes from a computer model by successively layering thin cross-sections of powder material. MPBF methods can produce extremely thin walls, 0.1 mm or less, making them very relevant for heat exchanger applications.
Copper
Copper is a potential material for heat exchanger design. Pure copper has excellent thermal conductivity, making it ideal for diffusing heat in various applications — from microelectronics to injection molding tool inserts. However, this property makes maintaining a neatly defined melt pool complex in MPBF processes.
Furthermore, the material’s reflective properties make the laser sintering of copper powder challenging with red light lasers, which are used in almost all metal AM systems. You can overcome these challenges by using the right design engineering software and carefully selecting your additive manufacturing method.
Aluminum
Aluminum is an ideal material for heat exchanger components due to its high thermal conductivity and low density. These properties make it suitable for applications where weight is critical, such as in aircraft or spacecraft. In addition, aluminum has a high strength-to-weight ratio, making it well suited to applications requiring high strength at elevated temperatures.
Finally, aluminum is highly resistant to corrosion, making it appropriate for harsh environments. Innovative heat exchanger designs can utilize aluminum instead of heavier metals to achieve the same heat transfer at a potentially lower weight and reduced cost.
How additive manufacturing improves HEX designs
You can utilize additive manufacturing to create exceptional HEX designs.
Optimizing lattice structures
Lattice structures are commonly found in nature. The honeycomb pattern in beehives and repeating patterns in tree trunks are just two examples of lattice structures. These structures utilize the space around them to create complex shapes that traditional manufacturing methods often cannot fabricate.
Lattice structures are used to design the core of heat exchangers. The layer-by-layer manufacturing approach of additive manufacturing enables you to create lattice structures within designs that are highly complex with small features. These design features have a large surface area, high stiffness, energy, absorption, and porosity and can be manufactured cost-effectively with additive manufacturing.
There are multiple types of lattice structures. The most effective lattice structures for heat exchanger design are TPMS lattices, which offer many benefits. TPMS structures naturally separate two-liquid flows, provide a large surface area for heat transfer, and conform to the available design space. Additionally, these structures are self-supporting, easily manufacturable, and naturally separate the flow into two domains.
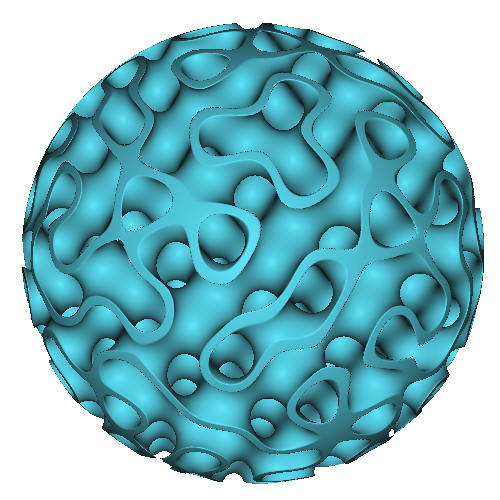

The gyroid TPMS (top) and diamond TPMS (bottom) are the most common structures used to fill the core of an AM HEX.
There are two standard TPMS cores: gyroid and diamond. These heat exchanger lattice core structures are effective in different applications. Before choosing a structure, you should consider various factors, such as operating temperature, weight requirements, available design space, and production cost.
Beam-based lattices can be useful when transferring heat from a solid to a liquid or air.
Field-Driven Design
The “classical” approach to simulation involves creating a design and then running a simulation to analyze it. This process was reactive, and you couldn’t generate geometry directly from these simulations.
With advanced engineering design software, you can now use simulations as an input that drives key variables to generate your design. This involves inverting the classical approach to simulation by first running a simulation to reveal the flow direction inside your heat exchanger and then creating a geometry around it. You can achieve this by leveraging a field-driven design approach.
This field-driven design approach represents a better way to generate and control complex part geometry, enabling you to control design parameters at every point from simulations.

Thermal simulation in nTop of Cobra Aero’s combustion engine cylinder heat sink. The thermal analyses results are driving the thickness of the lattice beams.
Lightweighting
Lightweighting is another goal of many HEX designs, especially in industries such as aerospace. However, you should tread lightly as their heat exchangers still need to perform in any end application. Additive manufacturing allows you to reduce weight while preserving overall strength and structural integrity. This means heat exchanger designs can perform in demanding environments.
Design time
Your team is likely under more pressure than ever to develop better-performing products in less time. However, you may struggle to develop adequate additive manufacturing designs in a reasonable time if you use outdated software. Manual processes can slow the design process while other teams wait for the HEX design to be finished.
Design software for AM heat exchangers
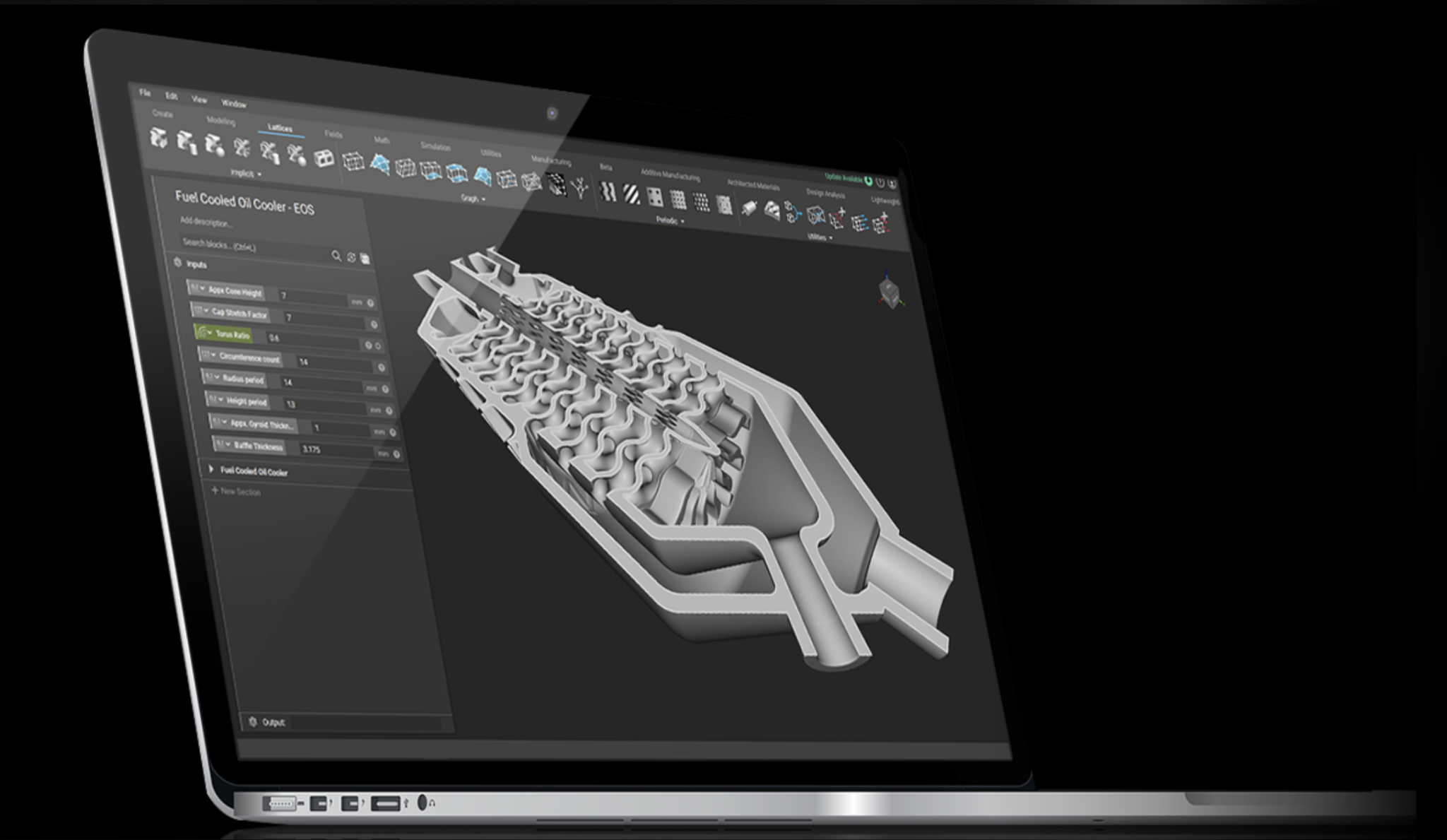
An advanced heat exchanger design in nTop.
If you’ve attempted to develop complex HEX cores using traditional CAD solutions, you have likely run into some issues. To enable you to develop innovative and complex heat exchangers, you need a software with the following capabilities:
- Includes tools that allow you to control TPMS and lattice structures
- Connects with your CFD simulation tools for verification or has built-in simulation tools
- Allows you to use CFD and thermal FE simulations to drive your designs
- Allows you to make design changes in a robust, reliable, and reusable way to speed up your iterative design process and make the development of AM HEXs cost-effective
Manufacturing heat exchangers with 3D printing is the future. nTop's engineering software enables you to produce compact, high-performance heat exchangers while maximizing the benefits of additive manufacturing.
Key takeaways
- You can improve heat exchanger design by maximizing heat transfer, minimizing pressure drop, and reducing weight.
- Heat exchanger design can be improved through lightweighting, incorporating lattice cores, and using test simulation results.
- The appropriate design software enables you to take advantage of additive manufacturing to optimize heat exchanger performance.
nTop
nTop (formerly nTopology) was founded in 2015 with the belief that engineers’ ability to innovate shouldn’t be limited by their design software. Built on proprietary technologies that upend the constraints of traditional CAD software while integrating seamlessly into existing processes, nTop allows designers in every industry to create complex geometries, optimize instantaneously, and automate workflows to develop breakthrough parts and systems in record time.
Related content
- WEBINAR
Eliminate Manual Meshing for Faster Heat Exchanger Simulation
- CASE STUDY
Replacing spacecraft supermaterial with high-performance lattice
- VIDEO
Creating a computational design workflow to lightweight drone panels with nTop’s new ribbing tools
- WEBINAR
Accelerating Product Engineering with Computational Design
- VIDEO
nTop Siemens Energy at CDFAM NYC 2024