Lightweighting with cellular materials: Insights from nature

Written by Dhruv Bhate | Associate Professor, Arizona State University
Published on August 21, 2020
Can cellular materials serve as a design solution for achieving lightweighting of engineering structures? Let’s listen to what nature has to say.
I know what you are thinking: cellular materials and lightweighting? Who made this guy a Professor? I hear you. While my students and I have spent a lot of time the past four years studying cellular materials, it has been in the context of energy absorption, honeycomb panel design, capillary action, and thermal management, and not with the intent to lightweight (is it now ok to use it as a verb?) a structure. However, when nTop approached me with a request to contribute to a series of talks on lightweighting, I wondered if this was a good time to enter the dark dungeon and chat with the trolls. The result of my explorations is this blog post, and the accompanying webinar, that I hope will spark some ideas, if not justify my hiring.
Traditional approaches to lightweighting involve material selection, consolidation, and topology optimization (Figure 1). Design solutions are of particular importance when working with higher density metals where composition is selected for reasons such as high-temperature performance or corrosion resistance, and the door to lower-density materials is closed. Cellular materials such as lattices and foams are typically not thought of as leading design candidates for lightweighting, and skepticism in this area is well-founded. There are several reasons for this, most fundamentally perhaps being the point that the very requirement of structure to have cellularity is in itself a limiting constraint from a design optimization standpoint. Nonetheless, I would like to stick my neck out and examine if this always must be the case.

Figure 1: Traditional approaches to lightweighting include (a) material selection, (b) consolidation, and (c) topology optimization. [Photo credits - (a): CES EduPack 2018, Granta Design Ltd., (b) and (c): nTop.]
The first argument for why we need to consider cellular materials in the context of lightweighting, and one I will focus in this blog post, is the argument from nature. We do find cellular structures in biological structure (Figure 2), such as insect nests, turtle shells, bone, and sea urchin spines, where mass minimization is always an inherent requirement. In some cases, such as the beaks and bones of birds, lightweighting is critical. As the songwriter J.J. Cale put it, “Travelin’ light is the only way to fly.” Fortunately he gives us no details about how this is to be accomplished, which is why engineers like you and me still have jobs in this world, and care enough to write and read (?) blog posts.

Figure 2: Natural structures where minimizing weight is of importance, and also exhibit cellular material design: (a) Venation of a water lily leaf, (b) wasp nest, (c) trabecular bone. [Photo Credits: Wikimedia Commons (a) Laitr Keiows; (c) Neon].
This apparent paradox can be resolved by making the argument that there are (at least) three performance objectives, which when sought, strengthen the candidacy of cellular materials for lightweighting: surface and volume infilling, multi-functionality, and damage tolerance. We find these demonstrated in natural structure, but also in engineering applications that embody cellular materials for these reasons, including honeycomb core commonly used in panels. These insights can be leveraged to inform engineering design of lightweight structures, and implemented by leveraging a framework for cellular material design that consists of four concepts: discretization, periodicity, gradients, and hierarchy. These concepts lend themselves very well to study in the context of nTop’s equation-based implicit modeling platform (small p intended). One example of this is work done by one of my students using nTop to create different geometries to study the effect of aperiodicity (Figure 3).
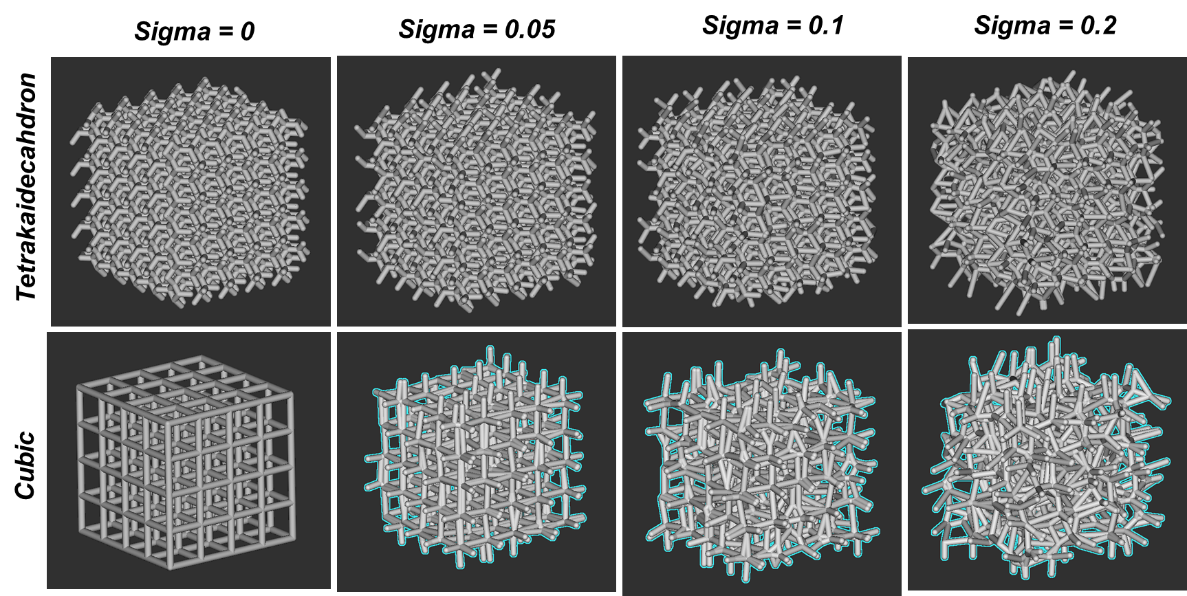
Figure 3: Use of nTop to develop cellular material designs with varying degrees of aperiodicity. [Photo Credits: Daniel Anderson, 3DX Research, ASU.]
Lest I be accused of “having a hammer and looking for a nail”, which while fair, is not the entire story, I would like to end by clarifying that cellular materials merely represent an alternative for lightweighting when the performance objectives mentioned previously are also being sought. However, I will go a step further and speculate that cellular material design is a critical element at the frontier of lightweighting since it helps us enable true multi-functional, material-and-structure co-optimization at a range of scales. Of course at this point, cellular design and other techniques blend into each other, and the boundaries between formal approaches to lightweighting start to break down. This is my second argument for why we need to consider cellular materials for lightweighting: they tie everything together. How am I so sure? Because life, uh, has found a way.
References
- Harris, “Five Techniques for Lightweighting: Doing More With Less,” January 2020, https://www.techbriefs.com/component/content/article/tb/pub/features/articles/35824
- du Plessis, C. Broeckhoven, I. Yadroitsava, I. Yadriotsev, C. Hands, R. Kunju, D. Bhate, “Beautiful and Functional: A Review of Biomimetic Design in Additive Manufacturing,” Additive Manufacturing, Volume 27, pages 408-427, May 2019
- Bhate, C. Penick, L. Ferry, C. Lee, “Classification and Selection of Cellular Materials in Mechanical Design: Engineering and Biomimetic Approaches,” Invited paper, Special Issue on Advances in Biologically Inspired Design, MDPI Designs, 3(1), 19, 2019
- J. Cale, “Travelin’ Light”, Troubadour, Shelter Records, 1976
- Malcolm, “Jurassic Park,” Universal Studios, 1993
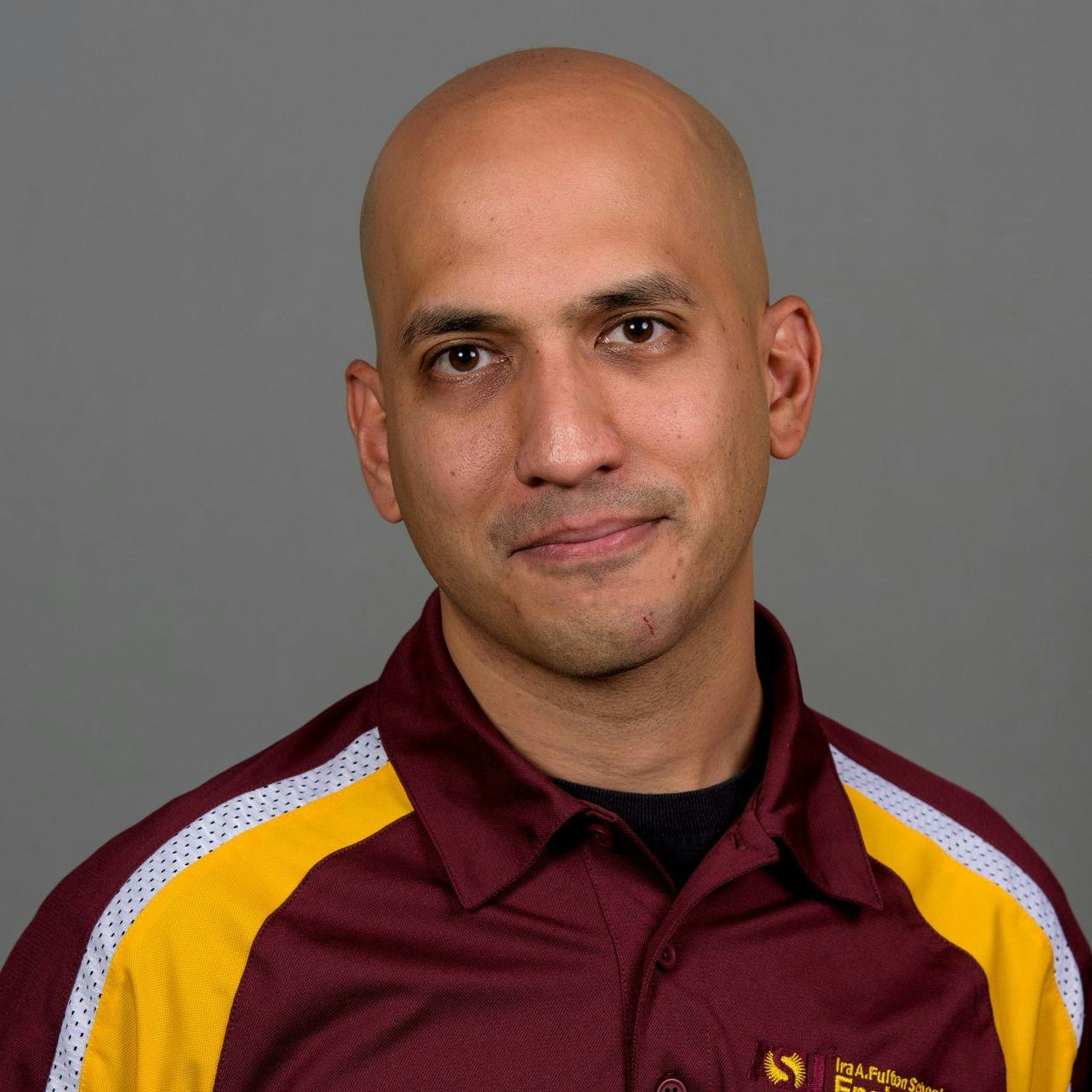
Dhruv Bhate
Associate Professor, Arizona State University
Dhruv Bhate is an Associate Professor in the Polytechnic School at the Arizona State University (ASU), where he leads a group of student researchers studying questions at the intersection of additive manufacturing, cellular materials, and bio-inspired design. He also teaches four different courses in manufacturing. Prior to joining ASU in 2017, he spent a decade in the semiconductor and additive manufacturing industries. He has a PhD in Mechanical Engineering from Purdue University.