Personalized medical device design: Complete guide
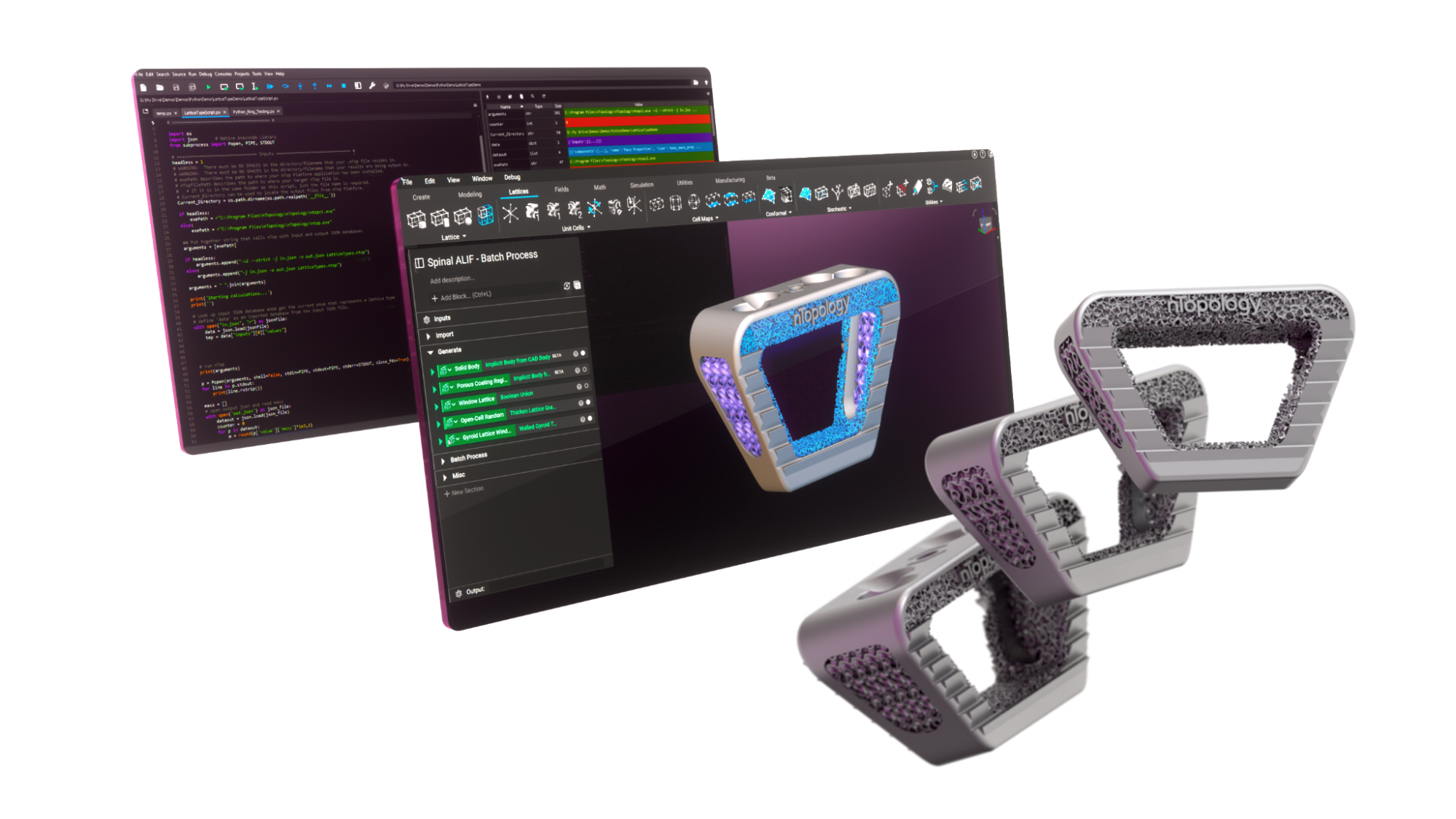
Written by nTop
Published on January 3, 2023
Personalized medical device design unlocks new opportunities to improve patient outcomes, reduce surgery times, and reduce costs. With the right tools, you can automate aspects of the medical device design process to minimize error and shorten total design time.
Applications
Key Software Capabilities
- Design automation
Introduction to personalized devices
Clinical studies have shown that personalized medical devices can improve patient outcomes and reduce the cost of care, making them an attractive option for healthcare providers.
While the basic concepts of personalized medical devices are not new, the convergence of the latest technologies enables companies to develop these devices in an increasingly cost-competitive and scalable way.
To make the most of these technologies, you must overcome various challenges — including additively manufacturing materials for medical device design, automating design, and complying with regulations.

3D-printed arm prostheses concept designed by Jade Myers in nTop.
Personalized medical device types
All personalized devices are made to order for a specific patient, but there are two main types of personalized devices to choose from depending on the regulatory environment and the use case.
Custom devices
These devices are custom-made according to a physician’s order to treat unique conditions that no other available device can address.
Patient-matched devices
These devices include customizable design features, with predetermined limits, to address a patient’s specific condition and needs.
From a product development standpoint, patient-matched devices are a more attractive option because their design and manufacturing can be streamlined and automated. This makes production economically viable at a mass scale.
Additive manufacturing for medical device design
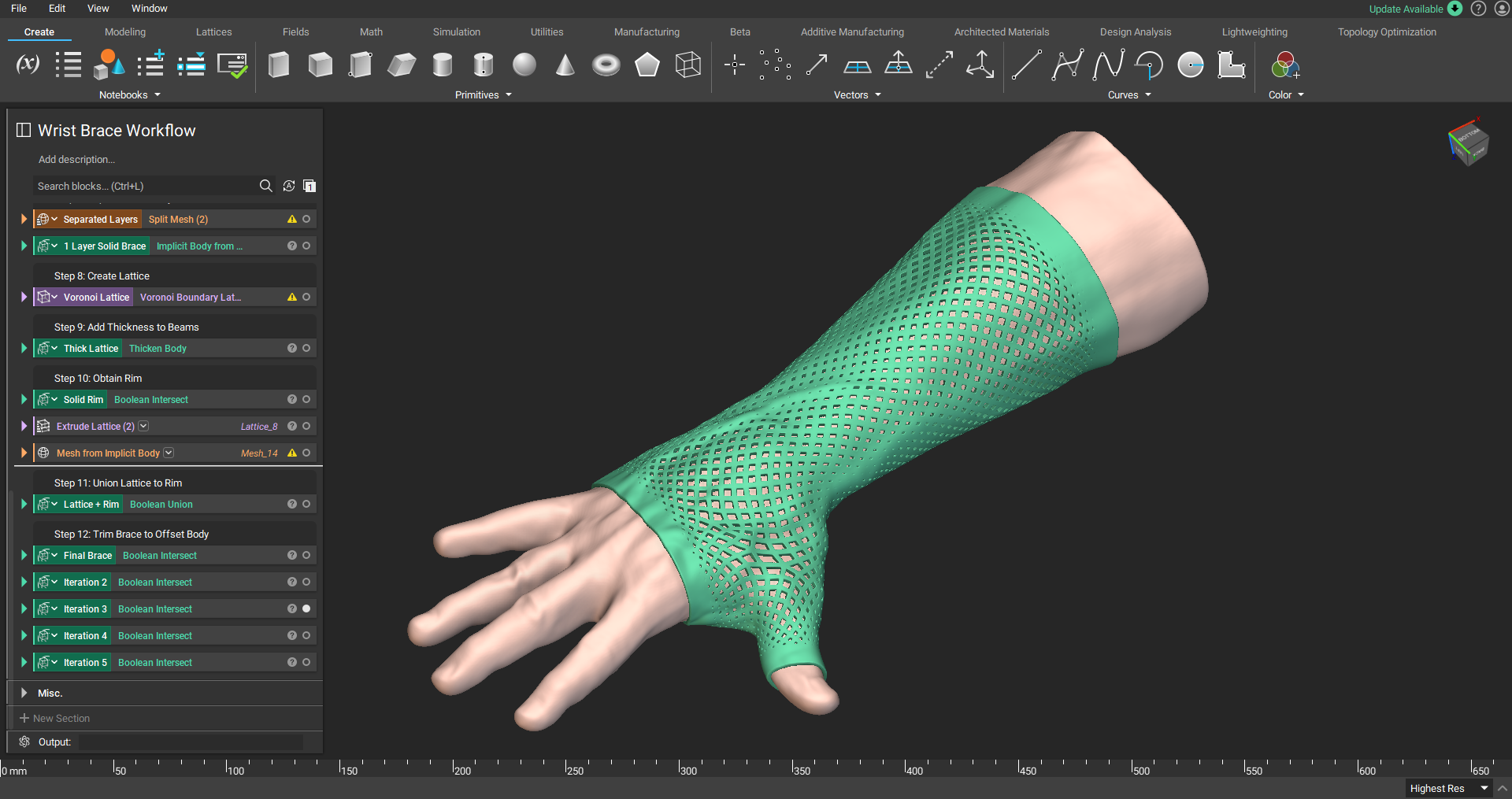
Patient-specific wrist-hand orthosis.
Additive manufacturing, also known as 3D printing, enables you to create personalized medical devices with features that would be impossible to create with traditional manufacturing technologies. These features allow you to create personalized medical devices that are economically advantageous and viable.
However, because the range of additive manufacturing materials available is limited, they can present a challenge. Fortunately, intelligent design and embracing additive manufacturing's ability to create complex structures can help you overcome this limitation.
Biologically relevant additive manufacturing materials
The medical field's most commonly used additive manufacturing processes include metal and polymer powder bed fusion, material extrusion, and vat photopolymerization. Desired material properties, device requirements, accuracy, and cost are critical for determining the best manufacturing process. Available biocompatible materials include PEEK, Titanium, and Nylon, but the range of biocompatible and sterilizable materials is still limited and is an area of active research.
Architected materials for medical devices
When a medical device application has material requirements not fulfilled by the relatively limited range of materials available for additive manufacturing, you may need to vary the physical response of those materials.
Fortunately, additive manufacturing allows you to create highly engineered structures that benefit from targeted performance characteristics. These structures are known as architected materials, and they enable you to treat specific physical properties, such as impact absorption or stiffness, as design variables.
Applying architected materials to your personalized medical devices can generate structures that mimic biological organisms, leading to better treatments. With architected materials, you can modify a design to allow for improved osseointegration, better static performance, or increased dynamic response.
Typical use cases for architected materials — including osseointegration, mechanical biocompatibility, and 3D-printed foams — are outlined below.
Osseointegration
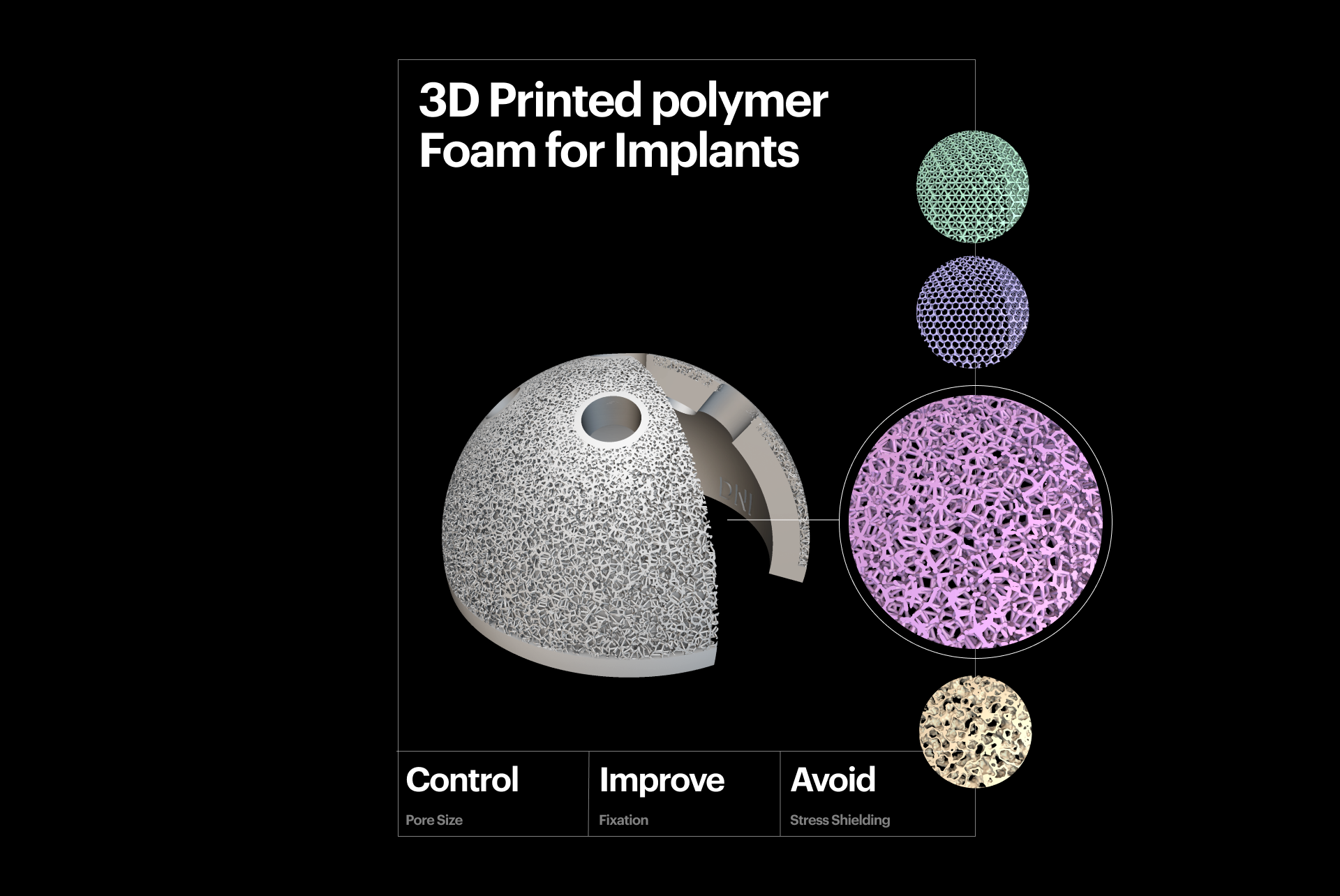
3D-printed polymer foam for implants.
Osseointegration, defined as a direct functional and structural connection between living bone and the surface of an implant, is crucial for implant stability. It is a prerequisite for implant loading and long-term clinical success.
Porous metal foams are a common feature of additively manufactured implants. Unlike traditional coatings, the attributes of these foams — such as pore size, porosity, distribution, and shape — can be fine-tuned to accelerate osseointegration. This is the case in both in vivo and in vitro clinical studies. These types of implants are often described as "cementless" and have been proven to reduce operating time by 25%, minimizing the risk of complications and saving healthcare providers time.
Mechanical biocompatibility

3D-printed trabecular structure.
A key aspect of creating personalized medical devices is taking steps to reduce the likelihood of "stress shielding", which occurs when metal implants are used to repair fractures or in joint replacement surgery. Stress shielding can lead to long-term complications for the patient. To mitigate stress shielding, you can fine-tune the mechanical properties of the implant, like compressive strength and Young's modulus, to match the bone's characteristics.
TPMS, graph, and stochastic lattice structures are excellent candidates for generating porous metal scaffolds, as an alternative solution to autologous bone grafts. This is because their characteristics can be optimized locally and precisely tuned to create the desired effect and mitigate the risk of implant failure. Additively-manufactured 3D scaffolds combined with cell-based treatments show promising early clinical results in oral and maxillofacial surgery.
3D-printed foams

3D-printed polymer foam for prostheses.
You can precisely tune polymer lattices to generate structures that offer foam-like behaviors. Unlike traditional foams, additively manufactured foams provide more control and excellent cushioning and impact absorption properties. In medical device applications, 3D-printed foams are often used in prostheses to create sockets that offer unique and dynamic behavior. This tailored approach improves the implant's performance and patient outcomes.
Design automation for medical devices
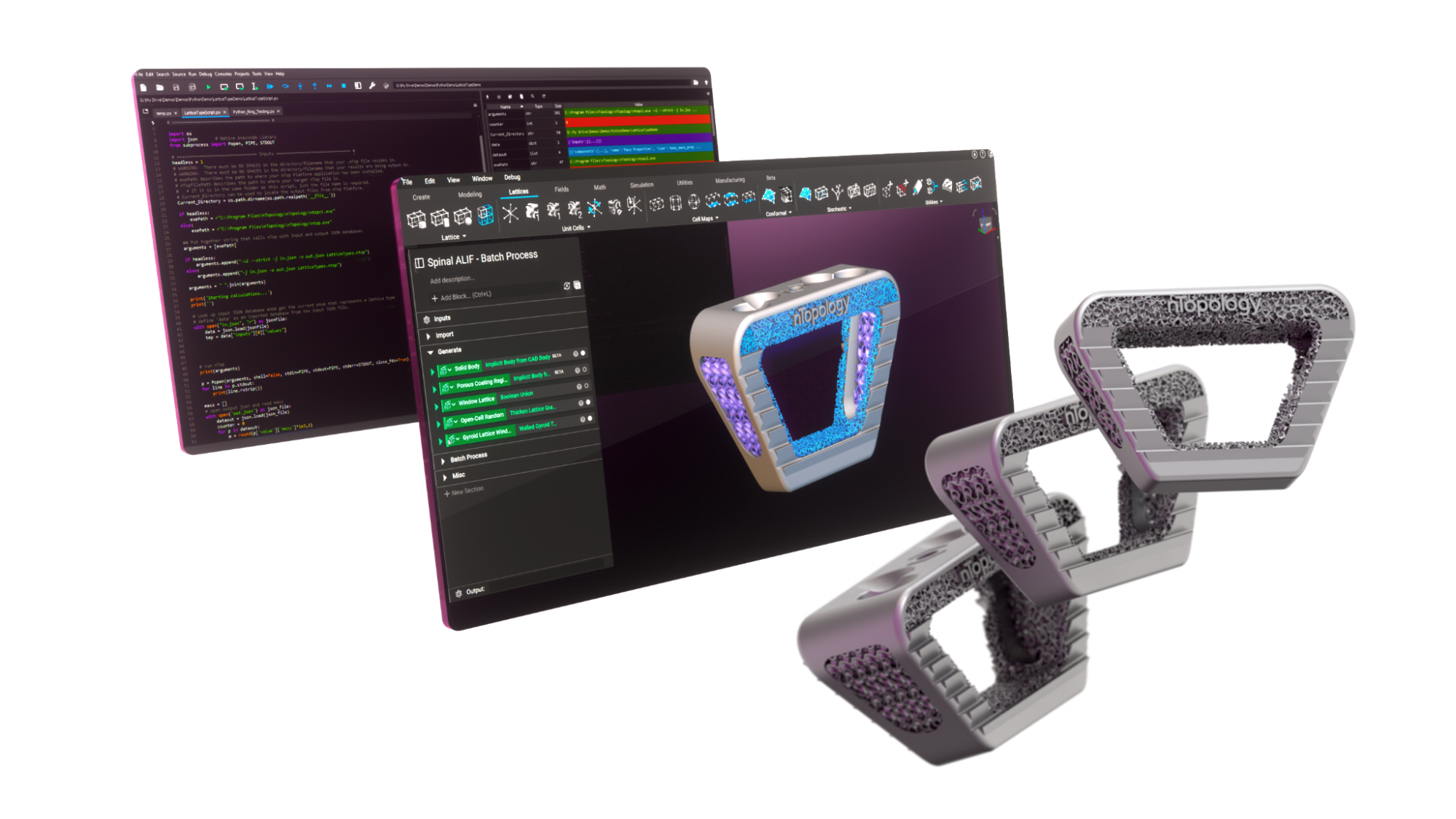
Design automation in nTop.
Customization costs during the additive manufacturing design phase can be considerable. This is another challenge you must overcome when creating personalized medical devices. It can take a minimum of a few hours to a day of work for a skilled engineer to manually design a personalized device, which contributes to higher costs and lead times. A high degree of design automation is essential for a personalized medical device to be financially viable.
By embracing design automation during the product development process, you can:
- Dramatically reduce personalization costs
- Shorten the medical device development cycle
The stages of product development are outlined below, showing where design automation is beneficial.
Development
In the research and development (R&D) phase, design automation allows you to explore the available design space quickly, identify key design variables, and lock in critical parameters. You should have an automated design workflow in place by the end of the R&D phase. Going forward, this workflow will enable you to rapidly generate a pre-validated design simply by providing new inputs.
Deployment
Once the workflow is set, you can deploy it in your production environment. At this stage, a non-engineer, like a lab technician, should be able to use a Graphic User Interface (GUI) to create new designs confidently. This allows your engineers to commit their attention and time to more complex tasks.
The GUI allows users to run automated design processes with patient-specific data as inputs, visually inspect results, and troubleshoot problems as necessary.
Scaling
Once your production volume has reached a critical level, you can use design automation via a programmatic environment to improve economic feasibility. For instance, you can use scripts running on a server or in the cloud and leverage design generation workflows to reduce manual design time and the associated costs.
Below are examples of how you can use design automation to reduce costs, accelerate the development cycle, and streamline operations.
Batch processing

Implantable orthopedic devices by SI-BONE, designed in nTop.
SI-BONE is a medical device company that specializes in minimally-invasive joint surgery. The engineering team at SI-BONE automatically applied an osseointegrative structure to a product daily with more than 70 unique parts. They achieved this in under six hours, all while ensuring traceability.
Build preparation

Croom Precision Medical’s build preparation.
Croom Precision Medical, a medical device manufacturer registered with the FDA, developed an automated design workflow that covered design, support generation, and slicing. The high level of automation means that users only need to tweak a few variables to automatically generate slice data ready to be imported to machine-specific software.
Data-driven design
Driving design variables with patient-specific data enables you to create personalized medical devices tuned to each patient's unique physiology. This data can be derived from simulation or direct measurements. As the two examples above show, design automation is essential in scaling and streamlining design operations.
Regulatory compliance in personalized medical devices
Regulatory approval, which might include FDA approval, is necessary to improve patient outcomes and ensure patient safety, but receiving regulatory approval can be an arduous process.
In addition to the usual validation hurdles, meeting regulatory requirements for personalized devices involves an additional challenge — you must also adhere to the same quality control regulations even when the geometry changes to accommodate personalization to different patients.
In practice, this means that when your device includes features that can be adjusted to the patient’s anatomy, you must ensure that these features can only vary within a pre-determined design window. During the qualification phase, you must prove to the relevant regulatory body that all your devices are within these pre-determined specifications (i.e., tolerances, material strength, defects, etc.).
Fortunately, certain regulatory bodies are now accounting for technological advances and granting clearance to innovative medical device companies for their patient-matched devices. This trend sets a precedent for future applications and improves the adoption rate of personalized medical devices.
Traceable design processes
A traceable design process can help you overcome the challenge of receiving regulatory compliance for your personalized medical devices. With a traceable design process, there is a digital trail for every design decision you make and for the input parameters that define your product.
A traceable design process is required by regulatory bodies to ensure that your submission passes review. It also improves your product's credibility, mitigates development risks, and allows you to figure out appropriate corrective actions in the event of error.
Design for traceability best practices
Implementing traceability best practices from the beginning of the design process can help you put your best foot forward when applying for regulatory approvals.
Here are some important traceability best practices.
Unique file naming
Using unique file names helps you track design variations across product development and production.
Design meta-data
Exporting key metrics and metadata for your design inputs and outputs as a text file is an important step in maximizing traceability.
Marking and serialization
By adding marks or serial numbers to your product, you can better identify the physical device when you go to production.
Test coupons
Automatically generating test coupons for quality control and validation eliminates a manual design step.
Design software for personalized medical devices

nTop is a next-generation design software built to enable engineers to take full advantage of the design freedom of additive manufacturing.
nTop gives you access to design tools that can help you overcome the challenges associated with developing personalized medical devices, enabling you to improve patient outcomes and establish a clear pathway for personalization at scale.
Find out more about personalized medical devices in our comprehensive guide.
Key takeaways
- Additive manufacturing offers superior design freedom, enabling you to create personalized medical devices with features that would be impossible to create with alternative technologies.
- To make the most of additive manufacturing, you must overcome various challenges — including additively manufacturing materials, automating design, and complying with regulations.
- With nTop, you can maximize the design freedom associated with additive manufacturing and overcome the challenges of automation and compliance.
nTop
nTop (formerly nTopology) was founded in 2015 with the belief that engineers’ ability to innovate shouldn’t be limited by their design software. Built on proprietary technologies that upend the constraints of traditional CAD software while integrating seamlessly into existing processes, nTop allows designers in every industry to create complex geometries, optimize instantaneously, and automate workflows to develop breakthrough parts and systems in record time.